As we look to transition away from fossil fuels, solar and wind are attractive options. Key factors making them compelling are: the inexhaustibility of the source with use (i.e., renewable); their low carbon footprint; and the independence that small-scale distribution can foster (I’ll never put a nuclear plant on my roof, even if it would make me the coolest physicist ever!).
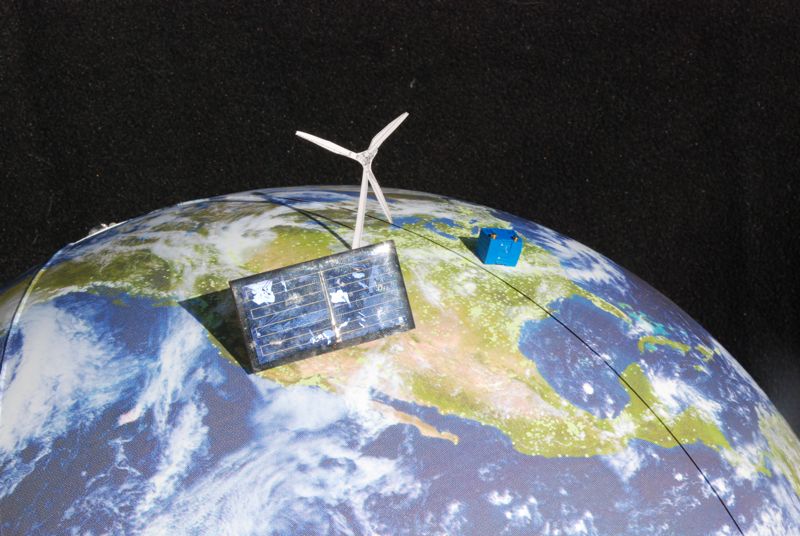
With full-scale solar in the desert southwest, and wind in the plains states, we're going to need a big battery (items not to scale!).
But solar and wind suffer a serious problem in that they are not always available. There are windless days, there are sunless nights, and worst of all, there are windless nights. Obviously, this calls for energy storage, allowing us to collect the energy when we can, and use it when we want.
Small-scale off-grid solar and wind installations have been doing this for a long time, typically using lead-acid batteries as the storage medium. I myself have four golf-cart batteries in my garage storing the energy from eight 130 W solar panels, and use these to power the majority of my electricity consumption at home.
It’s worth pausing to appreciate this fact. Compare this scheme to the dream source of fusion. Why do people go ga-ga over fusion? Because there is enough deuterium in water (sea water is fine) to provide a seemingly inexhaustible source of energy, and there are no atmospheric emissions in the process. Meanwhile, solar provides a source that will last longer (billions of years), produces even less pollution (no radioactive contamination of containment vessel), and is here today! It’s even affordable enough and low-tech enough to be on my roof and in my garage! People—we have arrived!
Storage works on the small scale, as many stand-aloners can attest. How would it scale up? Can it?
Meeting Requirements
So what would it take? We’re not a nation tolerant of power outages. Those big refrigerators can spoil a lot of food when the electricity drops away. A rule of thumb for remote solar installations is that you should design your storage to last for a minimum of three days with no energy input. Even then, sometimes you will “go dark” in the worst storm of the winter.This does not mean literally three days of total deprivation, but could be four consecutive days at 25% average input, so that you only haul in one day’s worth over a four day period, leaving yourself short by three.
So let’s buy ourselves security and design a battery that can last a week without any new inputs (as before this is not literally 7 days of zero input, but could be 8 days at 12.5% average input, or 10 days at 30% input). This may be able to manage the worst-case “perfect” storm of persistent clouds in the desert Southwest plus weak wind in the Plains.
Let’s also plan ahead and have all of our country’s energy needs met by this system: transportation, heating, industry, etc. The rate at which we currently use energy in all forms in the U.S. is 3 TW. If we transition everything to electricity, we can get by with 2 TW, assuming no growth in demand. Why? Because we currently use two-thirds of our energy supply (or 2 TW) to run heat engines, getting only about 0.6 TW out for useful purposes in the bargain. An electrical system could deliver this same 0.6 TW for only 1 TW of input, considering storage and transmission efficiencies.
Running a 2 TW electrified country for 7 days requires 336 billion kWh of storage. We could also use nuclear power as a baseload to offset a significant portion of the need for storage—perhaps chopping the need in two. This post deals with the narrower topic of what it would take to implement a full-scale renewable-energy battery. Scale the result as you see fit.
Lead-Acid Delivers
I’ll use lead-acid batteries as a baseline. Why? Because lead-acid batteries are the cheapest way to store electricity today. They’re bulky, sloshy, and very heavy, which makes them unsuitable for electric cars or laptop computers. But they’re very efficient, commonly achieving 85% or better energy efficiency in a charge cycle. The technology is well tested, having been around since 1859. And lead is a common element, being the endpoint of the alpha-decay chain of heavy elements like uranium and thorium. Their economic favorability makes lead-acid batteries hands-down the most common battery type in stand-alone renewable systems worldwide.
Large lead-acid batteries occupy a volume of 0.013 cubic meters (13 liters) per kWh of storage, weigh 25 kg/kWh (55 lb/kWh), and contain about 15 kg of lead per kWh of storage.
How do we put this into more familiar terms? A 12 V battery rated at 200 A-h (amp-hours) of charge capacity stores 2400 W-h (watt-hours: just multiply voltage and charge capacity), or 2.4 kWh. 200 A-h means that the battery could discharge a 10 amp current (120 watts) for 20 hours, or a one amp current (12 watts) for 200 hours—though in actual practice the capacity is lower at higher currents.
I can’t resist the temptation to ask: what is the minimum amount of lead that is theoretically needed to build the battery? The chemical reaction for a lead-acid battery is such that each interaction involving the transformation of one lead atom to PbSO4 liberates one electron at a 2.1-volt potential. This electron then is bestowed 2.1 electron-volts (eV) of energy, amounting to 3.4×10−19 J (see page on energy relations). One kilowatt-hour is 3.6 million Joules (1000 W times 3600 seconds), so that it takes 1025 lead atoms (where every one participates). If you remember that Avogadro’s number is 6×1023, we need about 20 moles of lead atoms. At 207 g/mol, this comes out to about 4 kg per kWh of energy, which is a factor of four less than the realized value above. Real implementations always fall short of theoretical ideals, so this isn’t new. We would do well to push for future improvements on this score, although we should bear in mind that lead-acid has had 150 years of development before we get carried away by dreams of perfection.
The National Battery
Putting the pieces together, our national battery occupies a volume of 4.4 billion cubic meters, equivalent to a cube 1.6 km (one mile) on a side. The size in itself is not a problem: we’d naturally break up the battery and distribute it around the country. This battery would demand 5 trillion kg (5 billion tons) of lead.
Get the Lead Out!
A USGS report from 2011 reports 80 million tons (Mt) of lead in known reserves worldwide, with 7 Mt in the U.S. A note in the report indicates that the recent demonstration of lead associated with zinc, silver, and copper deposits places the estimated (undiscovered) lead resources of the world at 1.5 billion tons. That’s still not enough to build the battery for the U.S. alone. We could chose to be optimistic and assume that more lead will be identified over time. But let’s not ignore completely the fact that at this moment in time time, no one can point to a map of the world and tell you where even 2% of the necessary lead would come from to build a lead-acid battery big enough for the U.S. And even the undiscovered, but suspected lead falls short.
What about cost? At today’s price for lead, $2.50/kg, the national battery would cost $13 trillion in lead alone, and perhaps double this to fashion the raw materials into a battery (today’s deep cycle batteries retail for four times the cost of the lead within them). But I guarantee that if we really want to use more lead than we presently estimate to exist in deposits, we’re not dealing with today’s prices. Leaving this caveat aside, the naïve $25 trillion price tag is more than the annual U.S. GDP. Recall that lead-acid is currently the cheapest battery technology. Even if we sacrificed 5% of our GDP to build this battery (would be viewed as a huge sacrifice; nearly a trillion bucks a year), the project would take decades to complete.
But even then, we aren’t done: batteries are good for only so many cycles (roughly 1000, depending on depth of discharge), so the national battery would require a rotating service schedule to recycle each part once every 5 years or so. This servicing would be a massive, expensive, and never-ending undertaking.
Who Needs Lead-Acid?
I focus here on lead-acid because it’s the devil we know, it’s the cheapest storage at present, and the materials are far more abundant than lithium (13 Mt reserves worldwide, 33 Mt estimated global resources), or nickel (76 Mt global reserves, 130 Mt estimated land resources worldwide). If we ever got serious about building big storage, there will be choices other than lead-acid. But I nonetheless find it immensely instructive (and daunting) to understand what it would mean to scale a mature technology to meet our needs. It worries me that the cheapest solution we have today would break the bank just based on today’s cost of raw materials, and that we can’t even identify enough in the world to get the job done.
This post does not proclaim that there is no way to build adequate storage to accommodate a fully-renewable energy infrastructure. A distributed grid helps, and an armada of gas-fired peak-load plants would offset the need for full storage. Storage can be augmented by pumped hydro, compressed air, flywheels, other battery technologies, etc.
Rather, the lesson is that we must work within serious constraints to meet future demands. We can’t just scale up the current go-to solution for renewable energy storage—we are yet again fresh out of silver bullet solutions. More generally, large scale energy storage is not a solved problem. We should be careful not to trivialize the problem, which tends to reduce the imperative to work like mad on establishing adequate capabilities in time (requires decades of fore-thought and planning).
Hits: 14948
Good analysis. I’d like to see a similar calculation for pumped-storage hydroelectricity.
Water in lakes has ~300k cubic kilometers ~ 3 * 10^14 kg
http://ga.water.usgs.gov/edu/earthwherewater.html
Potential energy is ~ 9.81*weight*height
Storage requirements (USA): 336 billion kWh = 1.2 10^18 J
height = 1.2 10^18 / (9.81 * 3*10^14) kg ~ 408m
We would need to pump water in all lakes about 408m high to have 7 day worth of reserves for USA.
I agree. When you are talking about nation-scale, certainly there are technologies that are much cheaper and can deal with near-infinite charge-discharge cycles (with proper maintenance).
Actually, there is no one-way to produce and store energy, in the future it’s going to be diversified. I don’t know if you were only trying to demonstrate that what we are doing with oil (one energy source only) isn’t sustainable, if it’s the case, good job. If you were trying to expose that we are doomed with renewable energy, than you are wrong in so many ways. We don’t need and don’t want a centralized energy source like Fusion or gigantic batteries arrays in the middle of a desert. What I want is to produce and store my own energy. How this energy is transformed and store is highly dependant of your location and your situation. I live near Montréal, Québec, Canada. We have a cold winter here so it’s not worth it to convert solar energy to electricity and then heat the house. Here, it’s better to use solar heated pipes with a fluid or geothermal heating. Moreover, there is planty of way to store electricity actually and there are also other technologies just around the corner. We could store the solar energy in hydrogen liquid (or solid, depending on the breakthroughs) form: during the day, when you are at work, your solar panels energy is used to separate oxygen and hydrogen from water and store it in a tank and do the reverse process when we need electricity. Electric cars could do the same thing by the way: usually in a week, cars are in a parking all day long doing nothing, they could recharge themselves (a bit) by doing the same H20 splitting process.
YoG, you are right on the money with your observation about diversification. Another way to put it is that in the absence of practically unlimited resources, people/families/communities must adapt their habits closely to the specific circumstances.
Emphasis on the general at the expense of the specific is one of modernism’s worst habits; it’s borne of abundance taken for granted, and it promotes wastefulness.
I do get kind of suspicious when I hear people talking about systems that do too much conversion of energy from one form to another. There’s considerable loss during each transformation.
Cool! Great post!
Do you really need that much stored energy? The example assumes the entire landmass of the USA is both dark and in a nation wide storm all the same time! I’m not from the USA but from Australia and the 48 states are *roughly* the same land mass as Australia. Now it’s extremely unlikely that a storm would cover the entire west coast and this is where distributed generation has a benefit – it’s not sunny here by 100 (or 1000) km away it’s blazing hot (similar argument with wind) so a bit simplistic…
In general storage is needed because renewables aren’t easily ‘dispatchable’ – that is the energy production in renewables (exception here is hydro which is one of the most dispatchable forms of energy) but if you flip the equation and factor in dispactchable demand (allow heating / air condition to fluctuate) then the system is far more dynamic on demand/supply. It would be good to here these factors in your comments.
Cheers!
Re-read the sentences about a more realistic interpretation of the “blackout” days. A 7 day “blackout” does not literally mean 7 days, or black.
I entirely agree with your scenarios for a single isolated node (I used to us solar arrays for remote telemetry equipment) but if you connect generating nodes (be they solar, wind, tidal, hydro) over a vast geographic area the local affects of storms and localised peaks and troughs can be somewhat smoothed out.
How about an analysis of what it would take to build one or more O’Neill colonies and bring space solar on-line? Beaming solar energy directly to Earth-based rectenna’s 24×7 would go a long way to eliminating the need for massive storage solutions. The original study talked about mining lunar material to build the powersats – but is that really practical? How much would be required and could it actually be done in space with any practicality? What about the orbital debris problem using the original mass-driver/catcher solution for getting the raw materials from the moon to the factory orbit?
An article on Ars Technica documented the net availability of wind power on the Eastern seaboard. http://arstechnica.com/science/news/2010/04/it-looks-like-time-to-build-an-atlantic-seaboard-wind-grid.ars
On the scale of the entire Eastern Seaboard, net average wind availability has a low variation. In principle, the total storage requirements would be far lower. Also, the price of batteries is high enough that such storage isn’t usually even on the table for large projects like this. The typical solution is backup power using combined-cycle gas turbine plants or other peaking sources. A typical data center scale UPS only has about 10-15 min of backup battery power, with the remainder supplied by emergency diesel generators. Why should the nation’s UPS be any different?
Good comment. The UPS with generator backup is a successful model that I rely on myself for my experiment in New Mexico. If we wanted to scale this up for the country, having an hour or even a day of battery storage built in, we would effectively need to maintain a substantial fraction of our current fossil-fuel electrical capability, despite a huge investment in renewable infrastructure (nuclear fission can help with baseload, but cannot dynamically react to intermittency). Yes, we would use less fuel (a great step), and the (skilled/trained) workers at the sites may have fun playing a lot of cards while waiting for the alarm to go off, but it would be a costly backup system.
The UPS idea is definitely more feasible than the (really impossible) nation-sized lead-acid battery. But most estimates I’ve seen claim that if we relied on more than about 20% of our power coming from intermittent renewable resources, the adaptability of fossil-fueled power plants to fill the gaps is compromised, and we need actual storage. I have not performed a similar analysis myself, but the results strike me as being plausible. I think there is near-universal agreement that we need genuine and significant storage solutions.
Interesting blog post, but:
1. You ignored the first part of Jonathans post: “On the scale of the entire Eastern Seaboard, net average wind availability has a low variation.”
So you need much less battery storage, not even counting UPS+backup power system.
2. Electric batteries are not sole energy storage. We have working pumped storage plant (http://en.wikipedia.org/wiki/Kruonis_Pumped_Storage_Plant , if you are curios enough).
As other commenter pointed out, if you wannt to store all needed energy by one solution (even pumped storage plant) its not archievable, but if we account that you need much less storage nationwide(or even world wide) than on isolated site and use more than one solution to store energy, that’s another math, ant it might proove to be possible 🙂
Good points. Keep in mind that this post explicitly explores one possibility, and makes no claim that adequate storage is impossible. There is, of course, a large uncertainty in the problem regarding what the mix of intermittent inputs would be. Wide distribution and a super-grid would help a lot. But if even one day per year the eastern seaboard wind was at a lull, for instance, you’d need something like a day of storage for the scale of that resource, which is already a daunting challenge. I just want to be careful about trivializing the problem. It’s too easy to wave off things that will in practice be extraordinarily challenging, which tends to reduce the imperative to work like mad on establishing adequate capabilities in time (requires decades of fore-thought and planning).
I’ve read recently about using molten salts for storage, particularly with solar thermal plants – would this be more effective than the large lead-acid battery you’ve postulated? And while you’ve addressed the availability of lead, what about the costs and energy requirements of mining and producing the lead? There are also the costs and energy associated with the production of H2SO4.
Great blog, this and the rest. It’s great to see a physicist’s science-based approach to these issues (having earned my B.S. in physics), which is often in sharp contrast to claims from the the economist camp’s “the market will solve it” approach (I work with economists). Keep it up!
Regarding the nuclear power plan on your roof, I’d advise against it. Did you see the news article about the young Swede who wanted to build a homemade nuclear reactor and was (briefly) arrested after he called the country’s nuclear agency to ask if it would be legal (thus raising all kinds of fears)? 🙂
Perhaps not for the roof but one of these would be wonderful for the backyard.
(PhysOrg.com) — Underground nuclear power plants no bigger than a hot tub may soon provide electricity for communities around the world. Measuring about 1.5 meters across, the mini reactors can each power about 20,000 homes.
http://www.physorg.com/news145561984.html
You should get a copy of RMI’s Reinventing Fire when it comes out (pretty soon I hear). They use UCSD as an example, by the way.
Also look at the advantages of geographic separation of intermittent resources. For example, see Archer, C. L., and M. Z. Jacobson, Supplying Baseload Power and Reducing Transmission Requirements by Interconnecting Wind Farms, DOI: 10.1175/2007JAMC1538.1. This substantially reduces the need for storage.
Also look at http://www.ausra.com/pdfs/ausra_usgridsupply.pdf for a 365×24 solution for the US based upon CSP+TES. The storage is thermal, not electric. I would take this one with a grain of salt, but it is still indicative of what is possible using TES.
The anti-correlation of wind and solar means that used in conjunction they provide a better solution than either alone.
Finally, Electric Vehicles (EVs) with Vehicle To Grid (V2G) technology help substantially. Consider the articles at http://www.udel.edu/V2G/Peerreviewed.html for example. EVs help even without V2G because they can pause charging when the wind blows or the sun shines, and pause when it is still or cloudy. V2G is actually demonstrated technology.
Great blog, saw a link to it a few days ago on slashdot and will be checking in regularly in future 🙂
On the topic discussed in this entry, I was wondering about a few things. The first one being, what would be the ideal storage ratio between batteries and capacitors when the next generation of carbon nanotube capacitors become economically available? It would seem to me that a system that combines both would be good, for several reasons; capacitors have far better burst capability in both charging and discharging than batteries do, which is great in a system where both supply and demand are variable and unpredictable. They look like they may become far cheaper than batteries in the future too. And of course batteries will be better for longer term storage.
Another thing I wondered was how much your estimate of required storage could be reduced by factoring in two related things. These are that while your scenario is great for a small isolated system, population clusters will likely always have more traditional chemical/heat based fallback solutions, which is related to my second point: a lot of current renewable energy research is going into renewable sources of traditional energy production types, eg. methane reclaimation from sewage, cattle slurry, energy from waste plant material, etc. These sources energy source materials can all be saved up and be used to provide base load, although far more easily, or at least economically, in clustered settings than in isolated systems.
Here is another thing to factor in. Efficient nations and US states use about half the kWh per capita of the inefficient US states. The 10 most efficient US states used 7,774 kWh per capita in 2005, compared to 13,947 for the 40 least efficient US states and the DC. The inefficient ones use 79% more power than necessary. If we were to adopt the policies, incentives, and regulations of the efficient states at the Federal level, and got all states to the efficiency level demonstrated by the efficient ones, it would represent a decrease in total power consumption despite rising population out through 2034 in one model. See http://www.killian.com/earl/images/ElectricityScenario.gif for a graph of this.
One also sees very industrialized nations across the world being efficient. For example here is the 2006 kWh per capita of several:
Switzerland 7,898
Japan 7,702
France 7,328
Korea 7,516
New York 7,367
California 7,212
Denmark 6,707
Netherlands 6,694
Germany 6,663
United Kingdom 5,774
The US could be more efficient, especially in transportation, but keep climate in mind too. Western Europe generally has a milder climate, at both ends, then most of the US, and less ubiquitous use of either heating or air conditioning.
The outright lack of lead was depressing. I was cheered to later learn of sodium sulfur batteries, and I’d wonder if there’s battery-like solutions we can do with iron or aluminum or other elements which aren’t super-nova limited. (Or, for lithium, burned-up-in-fusion limited.)
Use of backup gas generators needn’t mean continued fossil fuel dependence; surplus power can be used to synthesize hydrocarbons from biomass or atmospheric CO2, though I’d worry a lot about the efficiency both of generation and of burning, if fuel cells aren’t used. Or hydrogen of course, with its peskier storage problems but perhaps greater efficiency.
The 10 most efficient US states in 2005 (in kWh per capita of electricity) were VT, NJ, ME, AK, MA, NH, HI, NY, RI, and CA. Look up the cooling degree days for these states and then look up the kWh per capita for states with similar cooling degree days. You will find large discrepancies despite similar CDD. So no, climate isn’t a major factor.
You will find a much higher correlation with blue state vs. red state. That one doesn’t surprise me. Blue states are much more likely to have NG and electricity decoupling of profits from revenues, which is more of the more significant factors in encouraging efficiency.
That’s a lot of lead. Really large batteries may take advantages of cheaper ingredients. Sodium Sulfur for example. Not practical at small scale because you have to keep them hot.
Flow thorugh batteries where both ion species are in liquid state have much merit in terms of power cycles over the life of the battery.
Now if only eestor’s batacitor actually worked…
Perhaps a better way to store energy is not as electrical potential in batteries, but rather as chemistry. Much has been made of the hydrogen economy, but H is hard to store, and can be tricky to work with. In the longer run perhaps we can find a way to easily convert excess power to either methane or methanol. As long as we have a love affair with private transportation, there will be a market for methanol. Most gasoline engines can be modified easily to run on methanol.
The very least expensive storage, other than perhaps pumped hydro in ideal circumstances, is high temperature thermal storage using large insulated gravity-contained rock beds to store sensible heat at temperatures of up to 800C. Even after accounting for conversion inefficiency, as-constructed rock bed cost is an order of magnitude less expensive than just the raw lead for lead-acid storage of electrons. It is far less expensive than molten salt or any latent heat storage material. There is no worldwide shortage of rock.
As yet there has been so little penetration of electric grids by solar of any kind that storage has been a minimal issue. When days or weeks of storage are needed, rock storage will be very common. The CSP plant of the future will be a central receiver type, using air as the thermal transfer fluid, with a giant insulated rock pile at the base of the receiver tower. Conversion to electricity will be with conventional steam equipment, including standard HRSGs for the air/steam heat exchangers.
Since all of these technologies are decades old, it is shameful that they aren’t already our most common method of supplying electrical energy. It’s time to get serious about getting started.
[Editor’s note: this comment is way longer than what I’ll normally accept, but the content is too good to pass up.]
This is just the kind of calculation I love. There’s plenty of room for argument about whether we actually need to store the entire US energy consumption for a week, but this calculation gives us the right ballpark answer.
But I started to wonder about sodium-sulfur batteries, an emerging technology I’ve been really intrigued by. The disadvantage of NaS batteries is, you have to keep them hot so the sodium and sulfur stay molten. However, if you build them really big, this is no big deal. The advantage is very high energy density, plus they’re made from two of the most abundant elements on Earth.
I took the time to re-do your calculations for Na-S batteries.
Like lead, Na-S batteries produce about 2 volts of potential. But since the atomic weight of 1 Na + 1 S atom is about 1/4 the weight of a lead atom, so you get about 4x the energy density of a lead-acid battery:
http://www.wolframalpha.com/input/?i=2.1+eV%2Fatom+%2F+%2854+grams%2Fmole%29+in+kilograms%2F%28kilowatt-hour%29
I calculate 1.0 kg of Na+S per kwh of energy stored, compared to 4 kg/kwh for lead.
Real-world sodium-sulfur batteries have an energy density of 4.5 kg/kwh (3.5x better than lead batteries), so the theoretical advantage does carry over into the real world.
Na and S needed per kwh:
0.4 kg Na
0.6 kg S
To build your 360 billion kwh “national battery”, we need:
216 megatonnes S
144 megatonnes Na
World annual production of Na and S:
70 megatonnes S (minerals.usgs.gov/minerals/pubs/commodity/sulfur/mcs-2010-sulfu.pdf)
0.2 megatonnes Na metal (http://environmentalchemistry.com/yogi/periodic/Na.html)
However, the low production of Na metal is mainly because there’s no demand for it. It’s produced by electrolysis of NaCl: the chlorine gas is extremely useful, but the Na is less so: it’s usually converted to NaOH (caustic soda) and sold dirt cheap. Salt electrolysis plants could probably retool to produce more metal and less caustic soda: world chlorine gas production is over 40 million tonnes, and NaOH production amounts to about 60 million tonnes (http://en.wikipedia.org/wiki/Sodium_hydroxide), which works out to 33 million tonnes of Na.
Thus, with a little work, our current infrastructure can crank out a “national NaS battery” in about 5 years. (144 megatons sodium needed / 33 million megatons produced per year). There are economic issues to consider, but from a physics perspective, this is doable!
The comparable figure for lead batteries is 1300 years.
On further research, I see it’s not a simple matter to convert current salt electrolysis cells to produce Na instead of NaOH. But the point remains: because of the abundance of Na and S and their high energy density, production of a national battery using NaS technology is orders of magnitude easier than using lead.