I have a confession to make. When we moved into our current house three years ago, we had to sell our clothes dryer due to gas/electric incompatibility (happens every time we move!). So we lived without a dryer for three years, hanging clothes out to dry, and generally being frugal about washing vs. re-wearing clothes. Well, after several weather-induced trips to the laundromat this winter, we (or can I lay this all at my wife’s feet?) finally broke down and bought a used washer/dryer set on Craigslist. We’ll still let the sun dry our clothes 95% of the time, but have other options now.
Even though this little vignette does relate to the common Do the Math theme of low-energy lifestyles, the actual point of bringing it up is that the washer/dryer came from a house that had just been on display as a model for energy efficiency—including the washer and dryer. At the house, we met Jason Beckman, of Classic Residential, Inc., who had carried out many of the efficiency upgrades to the house. I thought it would be instructive to have him perform an energy audit at our home—especially a blower door test to expose ventilation issues.
As a bonus, after the nominal audit activities were over, I was able to spend some quality time with the blower door, doing extensive tests in virtually every room in the house. What I found was certainly instructive for me, and hopefully will be useful to a broad audience as well.
Drafts Prevent Suffocation
Air infiltration can be a major piece of a house’s thermal loss budget. We all know that “drafty” homes are cold in the winter. Yet obviously we need enough circulation to breathe. As reported in a previous post, one can find several different standards for air exchanges per hour. Modern construction aims for a “tight” house to register 0.35 air exchanges per hour. Effectively, this means that the rate of air infiltration (in m³/s or cfm: cubic feet per minute) divided by the volume of the living space should come out to something around three hours. Another site points to ASHRAE Standard 62.2, which uses a more targeted approach specific to a home’s footprint. For example, my home calculates to an ideal rate of 0.22 air exchanges per hour. I have also seen a building tightness limit (BTL) standard of 15 cubic feet per minute per occupant, which translates to 0.15 exchanges per hour for my situation. Just open the window a crack if guests stay over…
Blower Door Concept
A blower door is a fan that fits into a doorway, and seals against the doorframe so that a house may be pressurized (or depressurized) by the action of the fan. A two-channel pressure meter (manometer) monitors the difference between internal and external pressure, and also between internal and fan intake pressure. The latter maps to the rate of airflow provided by the fan.
In a typical test, the house is pressurized to 50 Pa (1 Pascal is 1 N/m² or about 0.00015 psi), and the airflow rate is measured. I think of it as being analogous to an electrical circuit: the pressure corresponds to voltage; the airflow is current; the ratio of pressure to airflow (voltage to current) is resistance—just as in Ohm’s law. So the goal is to measure the “tightness” or resistance to airflow of the house. For reference, 50 Pa is the amount of pressure difference one experiences by descending 3.5 m (~11 ft) down a hill, or “diving” under 5 mm of water. In other words, your ears don’t pop due to the change.
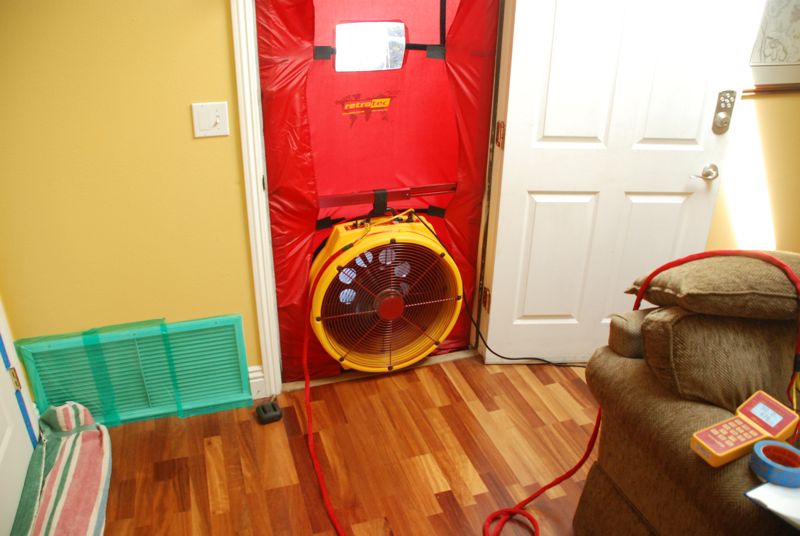
Blower set up in living room, in door to (open) garage. The sealed central air intake is seen at lower left. The manometer sits on the arm of the chair. The door to the rest of the house, at left, is taped all around (plus a towel for good measure). Different “range configurations” are available for the fan intake: here shown with eight equal holes open.
Let’s hang some numbers on the concept. The interior air space in my house (excluding walls) is 1540 square feet (143 m²). This makes for a volume of 12,300 ft³ (350 m³). When I pressurize my house to 50 Pa, I find a calibrated airflow of about 2530 cfm (cubic feet per minute; 1.17 m³/s). Divide these two numbers and we get 5 minutes. So this suggests 12 air exchanges per hour under a 50 Pa pressure differential. We could express the house’s resistance (in the tragic units of Pa/cfm) to be 0.02.
Because we only have two pressures at play, and all leaks between the inside and outside act in parallel, we will be better served by characterizing the conductance of the house: the inverse of resistance. In this case, my house has a conductance of 2530/50 = 50.6 cfm/Pa.
But the blower door imposes an extreme condition on the house in terms of differential pressure. How do we translate this into normal conditions? After all, the implied 12 air exchanges per hour is 60 times bigger than my ideal target of around 0.2.
Calibrating to Normalcy
The blower door asserts 50 Pa of pressure, and although this is only 0.05% of atmospheric pressure (~105 Pa, or ~15 psi), it is substantially larger than natural conditions tend to produce. And natural conditions vary from one place to the other. We’re really talking about wind here: it is the interaction of wind with a house that creates pressure differentials inside and outside of the house.
The Lawrence Berkeley Laboratory has defined a correction factor (PDF) by zone for the U.S. and Canada. The conversion factor tends to be around 20, implying that a natural pressure differential between inside and out is around 2.5 Pa. Interpreting this as a Bernoulli pressure or ram pressure for moving air: p = ½ρv², where p is pressure, ρ is air density (1.2 kg/m³), and v is velocity in m/s, we equate 2.5 Pa with a wind velocity of 2 m/s (4.4 m.p.h.), which seems perfectly reasonable.
For San Diego, a normally-shielded (by trees, structures) single-story house has a conversion factor of 24.5. It’s higher because San Diego is less windy than the average location. Let’s call it 25, so that my normal pressure differential is 2 Pa. Scaling down the 2530 cfm measurement, I would then expect a natural airflow rate of 100 cfm, amounting to about 0.5 air exchanges per hour. So my house is not nearly as bad as I expected: not terribly far from the tight building standard of 0.35 exchanges per hour (72 cfm for me), but still a factor of 2.2× the more targeted ASHRAE standard (45 cfm for me).
Thermal Impact
We care about limiting ventilation primarily for thermal reasons. We don’t want ambient air (hot or cold) infiltrating our house and defeating our efforts to stay comfortable. So how serious is a bit of leakage? For every 100 m² (1076 ft²) of floorspace, an air exchange rate of once per hour will require 85 W of power for every 1°C (1.8°F) of temperature difference between inside and outside. So if it’s at the freezing point outside and 20°C (68°F) inside, an air exchange rate of once per hour will require 1700 W to neutralize (per 100 m² of floorspace).
Putting this into perspective, the ugly theorist’s home modeled in a previous post with a floor area of 225 m² had a thermal loss rate of 280 W/°C when fully insulated. If this house has an air exchange rate of once per hour, we add 190 W/°C, nearly doubling the energy required for warmth (or significantly lowering the temperature inside if you, like me, don’t habitually heat your home). Cutting this by a factor of three to achieve the “tight” standard of 0.35 exchanges per hour results in air infiltration dropping from a 40% contribution to a 20% contribution.
So air leaks certainly can be responsible for a substantial fraction of thermal loss. For my house, the (measured) impact translates to 60 W/°C (72 when the fireplace damper is left open, as it had been for years). This is clearly an important thermal contribution, but not nearly enough to explain the horrendous (measured) heating requirement of 1465 W/°C for my house, as detailed in an earlier post. I had hoped that sticking a blower door on my house would allow me to account for the difference, and reveal the culprits that I could then remedy. I still have a mystery on my hands, but nonetheless managed to learn a bunch from the blower door experiments.
Blower Door Extravaganza
Getting a whole-house measurement from a blower door is a useful bit of information. But just as it is hard to decide on specific energy reductions by looking at a monthly energy bill, it is hard to know what the blower door test is telling us to do, without performing some breakdown.
Seeing how much fun I was having gathering blower door data, Jason was kind enough to give me some time alone with the blower door. We did it in every room. No—my wife is not jealous; accustomed, more like.
Room by Room
The first interesting test one can perform is to close all interior doors in the house to reduce the volume under test by the (front door) blower. Then opening up one door at a time provides an understanding of that room’s contribution. Because the blower senses and maintains a constant pressure difference, the airflow through any given leak is not altered by the opening or closing some other part of the house. In what follows, I will quantify each room’s conductance in units of cfm/Pa (straight division of cfm read off the meter by the 50 Pa operating point). Adding conductances for every room should match the whole-house measure. Since the whole house measured 2530 cfm, the total conductance is 50.6 units.
I should note from the start that closing doors is an imperfect sealing technique. Gaps around the edges (especially the base) can let a substantial amount of air whistle through. In fact, I found that simply closing the door can still let 50% of the airflow get through. Putting a towel at the bottom of the door can knock this down to 20% flow. But in my tests, I taped around all four edges of each door with painter’s tape to be sure I cut off the room entirely. I was amazed at how much air could move through a doorjamb gap even when it looked reasonably snug by eye.
The other necessary preparation for this room-by-room test is sealing off all central heating/cooling vents and intake. Otherwise the rooms are still connected by unseen tubes, and sealing off a door is wasted effort. Technically, one vent or one intake could be left open, but we’ll get to that later.
Below is a table of the separate partitions in my house and how much conductance I found for each, along with some indication about what makes that room have the characteristics it does. Cans refer to can lights. Doors refer to external-access doors.
Section | Area | Features | Conductance |
Kitchen/DR/Hall | 29% | fireplace, 7 cans, hood/vent, attic access, 2 windows | 17.8 |
Master Suite | 28% | double door, 8 cans, two vent fans, 2 skylights | 11.8 |
Living Room | 24% | sliding door, door to garage, 10 cans, skylight | 8 |
Guest Bath | 2.7% | vent fan, 3 cans, skylight | 5.4 |
Bedroom | 9.1% | 1 window | 1.6 |
Bedroom | 2.4% | 1 window | 1.2 |
Ducting | — | intake and 12 vents | 4.8 |
The common area connected to the front door in my house includes the kitchen, dining room, and hallway. Of significance, this space hosts a fireplace, a ventilation hood, 7 can lights in the ceiling, and three central air ducts. I measured a conductance of 17.8 (890 cfm at 50 Pa). Opening the fireplace damper added a whopping 11 units of conductance.
Opening up the guest bathroom (3 can lights, one exhaust fan, one skylight) showed its conductance to be 5.4 units. The master bedroom suite (two exhaust fans, 8 can lights, two skylights) displays a conductance around 12. The living room (10 can lights) has an intermediate conductance around 8. Two smaller bedrooms have relatively tiny conductances between 1–2 units.
The ducting for heating/cooling—though seldom used in my house— contributes nearly 10% of the air infiltration due to leaks in the ductwork.
So now I know where I stand room by room. This can help focus attention on the worst areas. But to really dig down, some nit-picky detail work needs to be done.
Really Room by Room
My next round of explorations involved setting up the blower door in individual rooms to measure their airflow in isolation of the rest of the house. The numbers were generally consistent with the whole-house approach, if not tending to be a little higher (suspect some leakage around blower foor frame). But the main advantage of narrowing the scope of the blower was that I could detect relatively small changes from sealing one thing or another.
Here’s what I learned, in a nutshell:
- Can lights average about 0.4 cfm/Pa apiece. Taken together, the 28 can lights in my house contribute 22% of the air infiltration.
- The central air ducting in my home accounts for 9% of the leaks. My ductwork is not particularly leaky. The energy audit turned up an 8% loss rate of forced air used for cooling/heating. Under 10% is considered good, and 6% is a “green” home target.
- Bathroom ventilation fans contribute an average of 1.6 cfm/Pa apiece. The three fans in my home contribute 9% of the air infiltration.
- A poorly-sealed stove hood duct (where it goes into the attic) contributes 2.7 cfm/Pa, amounting to 5% of our house’s loss.
- Electrical outlets and light switches average 0.03 cfm/Pa apiece. While this is a small number, my house contains about 75 instances, amounting to about 2.3 cfm/Pa, or 4.5% of the house.
- The attic access hole, covered with a slightly bent piece of sheetrock,
contributes 2 cfm/Pa, or about 4% of our house total. - The fireplace, with damper closed, still drew 0.9 cfm/Pa, making for a 1.7% house contribution.
Lots of nickel-and-dime stuff here. Adding it all up, I can account for over half of the air loss in the house. I suspect that the remainder is from doors, windows, plumbing entry/exit, and the odd gap hiding behind baseboards or other moulding. But the good news is that I have a list of action items that collectively could make my house twice as tight as it is. Throw on some better door seals and pay attention to plumbing entry/exit and I may even be able to accomplish a factor of three reduction.
Is it really so simple?
I’ve been treating my house like a big resistor following the oh-so-linear Ohm’s Law analogy. Does this assumption hold up? I did a mapping of airflow vs. pressure in steps of 10 Pa, finding that the relationship is sub-linear. In particular, I found an exponent of 0.66. For example, at one point I had tightened down my common area to 645 cfm at 50 Pa (started at 890; could have gone to 435 if I blocked all known offenders), then measured airflow at different pressures. I got the curve shown below, with a fit of 225×(P/10 Pa)0.66.
It was only after this that I understood one of the features of the manometer I was using for the blower door test. If the system could not achieve the pressure setpoint (due to excessive airflow for its range configuration), it would extrapolate to the standard value—usually 50 Pa. The user had a choice for which exponent to use in the extrapolation. From the manual:
n: sets the slope of the @-Pressure extrapolation line. Use 0.65 for tight houses and 0.60 for ducts and large buildings.
So there we are. Confirmed experimentally in Joe Random House.
What this means is that the factor of 20 (or 25 in San Diego) adjustment used to convert the 50 Pa test into natural conditions does not simply map to 2.5 (or 2.0) Pascals of inside/outside pressure differential in the linear way. Instead, we hit a factor of 20 in flow at a pressure of 0.53 Pa indoor/outdoor differential. The implied wind speed using a simple Bernoulli pressure is now down to about 1 m/s, but I’m not going to worry myself over what this means in practice. What I can say is that windier days will increase the indoor/outdoor pressure differential and promote more vigorous air exchange according to something like the graph above. The fact that the curve is steep at its beginning means that small changes in pressure/wind can have outsized impacts on airflow.
A Side Experiment
While checking an especially tight room, I had the blower at 50 Pa of pressure and only 125 cfm of flow. Then I opened the window to a 1-inch (25 mm) gap, 44 inches tall. The airflow soared higher by 550 cfm. At half that gap width, I saw a 340 cfm increase over the baseline. Half again and we’re at 255 cfm. Then I closed the window until I saw no daylight, but it was not pushed all the way into its housing against the seal: 85 cfm over the baseline. Roughly, we’re looking at a scaling law with an exponent around 0.55 as a function of crack width. This general curve shape is again indicating that gaps and cracks don’t act linearly: reducing the area of a gap by a factor of two or four reduces the flow not by the same factors, but by about 1.5 and 2, respectively. So attention to detail becomes important. The little gaps matter. This is also reflected in my finding that I had to tape all sides of a door, even if the gap looked tight, in order to knock out all the flow.
My Take-Away
I am simultaneously pleased and disappointed by the blower door results. In the plus column, I now have a measure of my house’s permeability, and can point to over 50% of the offenders, which means I can start knocking them off at will. Additionally, my house was not a drafty embarrassment by modern green standards, which I fully expected to be the case. On the down side, the source of almost half my air leaks remain mysterious—although I do have my suspicions. Also, the air leaks I measure cannot come close to accounting for the exorbitant heating/cooling requirements I previously measured for my house. The books remain open. One approach at this stage would be to seal up known leakers (at known impact), and repeat a heating/cooling test.
For my action items, I could retrofit my can lights with airtight trim units. Since we generally do not use our central air services, I could make sure all the vents are closed (even if imperfect) and even seal off the intake in an easily reversible way (substitute a piece of plywood for the air filter). Sealing the hood vent’s attic penetration is a quick and easy job. Likewise for the attic access. Updated door seals and stuffing old socks around plumbing penetrations may buy a little bit as well. I am less sure about finding bathroom vents that aren’t leaky. They usually have a flap that rests against the exhaust tube when the fan is off, but maybe better designs exist that are more completely sealed when not in use. And I don’t know if it is code-compliant to have a fully-sealed fireplace that is fitted with a gas line.
All the same, I have some work to do. And most rewarding: I have a sense for the sources and relative scales of airflow problems in houses.
Hits: 1627
Thanks so much once again for taking the time (oh, the time to compile this blog, I cant not begin to plumb the depths!) in not only doing the tests, and not only documenting the results, but then explaining the results!
My buddy and I have been talking about doing fan tests on our houses for a long time, your results are extremely interesting, there really is no silver bullet, its draft by a thousand tiny holes!
I always share your blog entries, my math head son and eco aware friends read and re-read them…. This one, even more so!
Great post, interesting results. I suspect my house behaves very similarly. In the past year I spent a lot of time sealing HVAC ducts, basement joist gaps and adding some padding to windows that were chronic drafters. I also spent ~$600 to add about 10-12 inch of blown insulation to my 1500sqft attic. I “think” the most obvious improvement has been the attic insulation. This winter has been much colder for much longer in MI, however I now keep the heat 1-2degrees higher than last year’s mild winter, the master bedroom (2nd level) is much warmer at night, and the gas bills are visibly lower. The utility gave me a $125 rebate for the insulation, and the feds gave me a $60 credit on my tax return this year. Not bad for a ~$450 investment which will probably rebound in 1-2 years. Now I wish I bought more insulation.
So for the can lights, do you just suck it up and buy LED retrofits? Those look like they would seal tight, if you were careful around the edges. Of course then you’re stuck with the brightness, efficiency, and color temperature of whatever you can buy today.
I saw a video online about airtight inserts that can still accommodate CFLs (what I presently have in all cans). I plan to look into this, as it would be the cheapest option for me.
Interesting tests. The latest building code in Denmark specify a maximum flow of 1.5 l/s per sqm for standard buildings, down to 0.3 l/s per sqm for energy efficient houses. If a you then get a leak in the vapor barrier at some hidden place, you may be in a lot of trouble during the winter when the warm and relatively humid air blows into the insulation material and condensate at the low ambient temperatures.
I generally prefer buildings that may be less well sealed.
Btw. surprised that you find a need for a dryer. Having lived in a cold and humid Nordic country for the past 5 years, we have managed to dry all of the family’s cloths outdoors (under a roof) – at times supplemented by a drying line in the garage during longer rainy periods. Perhaps you need a front loading washing machine with a proper centrifuge (+1600rpm)?
For comparison’s sake, 1.0 l/s in my house would translate to 1.5 air exchanges per hour: on the drafty side, and twice what I currently experience. The tight standard of 0.3 l/s is therefore 0.45 l/s in my house, so a bit draftier than the U.S. tight standard.
As for needing a dryer, I’m not going to disagree with you. Maybe my wife will read the comment and experience Euro-shame.
Have you seen the standards for passive houses?
http://www.passivhaustagung.de/Passive_House_E/airtightness_06.html
Such houses would have a heat exchanger to give air freshness while maintaining insulation. It’s an interesting comparision
Yes–these are great devices. I mentioned them in an earlier post. It would be difficult for me to get my house down to the point where I would have to provision airflow in this way. But for new homes built very well, it is a superb option.
It’s a perfect option! I’ve been living in a passive house apartment building for over a year now (the building is part of the largest passive house development in the world, actually), and the air quality and temperature is unlike anything I’ve experienced before. It feels like a light summer day all year long, no matter if it’s -15° or +30° outside. In winter, heat is recovered through the heat exchanger and on very cold days the air is slightly preheated (district heating); on hot summer days we use sunblinds to keep the heat out.
All in all, air quality and temperature feel completely “natural”. It’s a vast improvement over the radiator-heated/air-conditioned air you get in “normal” houses most of the time.
Be careful with the recessed (can) lights. Depending on age, they may or may not have a thermal strip that cuts them off when they overheat. New code rules have all sorts of requirements for keeping insulation away from the hot parts, or boxing out the units with firehats/sheetrock. You get into IC (in-contact) versus non-IC rated lights. Most older lights, and many new ones are non-IC.
In other words, don’t risk burning down your house.
Yes, most of my cans have no insulation right around them. As for making them airtight, I would worry about incandescent lights, but a CFL pumping out a whole 14 W is a lot less worrisome to me. Of course, I could retrofit with airtight inserts and never have a problem, then sell the house to some bozo who sticks in incandescents and burns the house down. So yes, there is reason to tread carefully here.
LEDs are even less worrisome when it comes to recessed cans.
Lack of insulation around can-lights and down-lights can reduce the overall insulation performance of a ceiling by as much as 50%! The other key areas where insulation fails is at the edge of the ceiling (around the perimeter of the roof) and near the top of walls or under wall studs where the insulation sags and leaves a gap.
A thermal imaging camera (or even a non-contact thermometer) is invaluable for finding these hot-spots/cold-spots. You will be amazed at what you find, even in a “good” house.
Cheers
I’m a little incredulous about the claim of 50%, but without knowing where this is going to go, let me figure out what might happen in my house. I have 28 can lights (a lot), and if a square foot were cleared of insulation around each one, about 1.5% of my ceiling would carry no insulation. The R-value of uninsulated ceiling is about 1.65 (U.S. units; see thermal house post for info). A nominal amount of insulation would make it R-13. The effective R-value would be 1.0/(0.015/1.65 + 0.985/13.0) = 11.78, or a 9% reduction. If we went super-thick on the insulation apart from the can lights (say R-40), then we’d get an R-value around 30. So a 25% reduction. Not trivial. I suppose one could stretch it to 50%, but this would translate to an excessive amount of can lights.
Thanks for instigating an interesting calculation.
Might this fall under the “The little gaps matter” discussion ?
Great posting as usual!
Also in San Diego, when we moved in to our house it was quite drafty. Found the first culprit with an infared thermometer – a missing damper over the stove. Next came the chimney damper (reg.s require a minimum small opening since there is a gas supply so it can’t be fixed entirely) and then the windows. The new windows took care of most of our drafts. Then I noticed the uninsulated garage was the next big factor as it bled tons of heat into the house through the walls in summer and reverse in winter. I had not anticipated the garage would be such a large thermal sink. I changed the big door to an insulated one and added insulation to the attic space of the garage which together modrated the problem. The place is much more livable now.
Nice experiment, and welcome back. I was beginning to worry you had blown this blog!
Many expressed concern for my disappearance, and I appreciate the sentiment. I became so utterly bogged down in my professional juggling act that I simply had to shed this (and other) elective activities for a while. Plus, I have emptied my brain on this blog, and have fewer topics available that I can expound upon without dedicating time to research first. So I doubt I will be able to get back to a solid bi-weekly cadence, but I’ll do what I can.
Tom, you’ve covered a lot of territory over the past year and it really is true – once you detail the physics of a situation, there’s not much to write about on that topic, since the numbers don’t change unless the laws of physics change – something most “techno-optimists” I engage with seem to have trouble accepting.
You have the basis of a very good book on this site. As a solar/renewable energy teacher, I hope you publish it someday.
“And I don’t know if it is code-compliant to have a fully-sealed fireplace that is fitted with a gas line.”
Here, code requires a bolt that keeps the flue open a minimum amount. We put an insert in the fireplace opening — heavy plastic film on a rigid aluminum frame with foam rubber sealing around the edges. It made an enormous difference in terms of drafts in the living room in the winter. We get regular high winds here with gusts up into the 40-50 mph range. The flexible film gives us a nice demo of the Venturi effect as the wind velocity across the top of the chimney varies.
Here in our sub-zero winters, code requires no damper at all, making our gas fireplace insert into a funnel of icy wind in winter that passes right through the gaps in the glass doors. We solved this problem as follows:
1) Cut a large piece of Reflectix foil duct insulation to exact opening size of the fireplace to fit snugly over the doors (a small slit was used for the door handles)
2) With metal duct tape, attached flexible 2″ magnetic strips around the edges of the foil facing the doors
The magnets adhere to the fireplace insert metal frame, preventing almost all air inflow. A little tug lifts the whole thing away when we want to use the fireplace.
Next problem: the masonry around the fireplace is icy cold since it is uninsulated. Solution?
I done some interesting experiments with my house related to your post. I live in Iowa which has cold and windy winters. During the day (6 am to 10 pm), I keep the thermostats set at 65 F. At 10 pm, they are turned down to 55 F. The house temp slowly drops until 6 am but rarely hits 55 F. I try to wake up about 5 am and record the inside temperature. I use the NOAA weather data for my town to get the night’s average outside temperature and wind speed. I can then calculate the thermal time constant of my house for that night. I have done this for a number of nights and can plot the thermal time constant versus wind speed. It is surprisingly linear with wind speed. In round numbers, my house has:
thermal time constant = 60 hours – (wind speed) * (1.5 hours/mph)
I assume that my house’s thermal capacitance does not change with the wind.
Therefore I have a measurement of how my thermal conductance varies with wind speed. Actually, the fit is only linear if the wind is from the West, NW, or North. I have found that East winds have much stronger effect negative effect on my time constant. I have not figured out the source of this yet, but I was surprised. I have really enjoyed your blog and it has inspired me to make measurements like this so I wanted to give back. Thanks.
A great experiment! Very interesting about directional dependence. What is your average winter wind speed? If it’s something like 10 mph, you could conclude that air infiltration accounts for about 25% of your thermal loss, which is an interesting number to know. Thanks for sharing your results!
Checking several sources suggests that 10 mph is a reasonable average winter wind speed for me. I will combine this assumption with some other measurements to give more results for my house.
After getting data from my natural gas company, I was able to fit the therms that I used each month versus the Heating Degree Days in F for the month. It was gratifiying to split this data into before and after adding insulation.
before insulation : .11 therms / (month * HDD_F) = 240 W/C
after insulation : .075 therms / (month * HDD_F) = 165 W/C
I have an older furnace that is probably 70% efficient.
So my house’s average thermal conductance is now about 115 W/C. From my original post, an average 10 mph wind speed gives an average thermal time constant of 45 hours. Combining these give the house thermal capacitance = 45 hour * 115 W/C = 5.2 kWh/C
Using the wind speed measurements in my original post, my house has:
thermal conductance = 5200 W/C / (60 – 1.5 * (wind speed in mph))
wind = 0 gives 86 W/C, wind = 10 mph gives 115 W/C
My house is 1800 ft2 with 8 ft ceilings giving 400 m3. Using air thermal capacitance of 1250 J/C/m3 gives my house’s air holds 500,000 J/C = 140 Wh/C = .14 kWh/C
A 10 mph wind lowers my thermal conductance by 29 W/C (= 115 – 86) which implies:
10 mph wind causes extra 29 / 140 = .21 air exchanges per hour
This must be an underestimate because some of this extra air is coming through the basement and garage so it is not at the outside temperature. I thought that you would be amused by these numbers.
We experienced our first winter in our passive house here near Paris. The overall electricity need here (which is the only power source, except for the sun), divided by the measured temperature delta (inside-outside), gives 110W/°C for the last 3 months (inside temperature approximately 19°C, minimum daily mean outside temperature was -5°C). This is calculated from measured instantaneous power requirement and continuous temperature measurements (all logged in RRD files).
The mean power requirement for the same 3 months is 1677W (for the whole house, not per m²…). The base power requirement is nearly 300W (refrigerator, computers, internet box, phones, various stand-by, etc.), which ultimately generate heat, which is good during winter. The same applies to electricity-heated water : there is no wasted heat here because it remains inside the insulated volume (except during a shower, for instance).
Nota : the tighness of our house was measured to 0,39 volume per hour, under 50 Pascal pressure. I was impressed by your first standardized figures about less than 1 air exchanges per hour, until I understood it is under nearly no pressure at all… which is less than unimpressive…
Great info, and especially appreciate the numbers!
One concern: I suspect that your house was measured at 50 Pa, as is standard practice, and then extrapolated down to natural conditions (also standard practice, at least in U.S.). It is possible that the person performing the measurement was unaware of the correction, if software intercedes. Otherwise you would be looking at a corrected natural rate of one exchange every 50 hours. This is well below the benchmarks for healthy, breathable airspace. Are you 100% sure about your number?
I’m pretty sure there is no correction on this side of the planet, even though software does the measurements and calculations.
This is the reason why this kind of house definitely need a mechanical ventilation unit with heat recovery. I suspect that air wouldn’t be comfortable if the unit stops for more than 1 day and we do not open the windows.
The added benefit of the heat recovery is that ventilation no longer leaks heat out of the house, which makes for the 110W/°C electrical input.
Nota : there is another strandard here in France, where the thightness measurement is made at 20Pa instead of 50Pa, the maximum tolerated value is roughly the same 0,5 volume per hour, with the tightness quality obviously much less (same leaks at half the pressure).
Late addition/edit to original post:
For reference, 50 Pa is the amount of pressure difference one experiences by descending 3.5 m (~11 ft) down a hill, or “diving” under 5 mm of water. In other words, your ears don’t pop due to the change.
Should you ever be able to get the blower door back, here’s a useful trick: set it to depressurize the house, then wet your hand and walk about looking for spots that chill your hand. It’s a good way to determine where the leaks are. Also, I was taught to depressurize the house when performing a blower door test. (This was back in the 90s!) The reasoning was that the depressurization would ensure the flappers on the dryer and exhaust fans would close. Pressurizing would tend to open all those flaps, giving an unrealistically high result.
Neat trick. I did walk around with a bit of tissue that would start to flap around leaks; even get drawn towards power outlets when held close. I also wondered about flappers, etc. But at least I have quantified the effects of each of those units. I was hesitant to depressurize as I thought I might bring unwanted dust inside from the attic and walls (insulation dust).
A lot of the time I want to criticize your blog for the constant US-centrism, but at the same time it’s teaching me a lot about the surprisingly large differences between the US and Scandinavian countries (I’m from Norway). I’ve lived my whole life without a clothes dryer, and couldn’t imagine the need for one until I befriended some americans last year who didn’t know what to do without one. I can just assume the weather is better suited in San Diego than up the cold north here, and judging from your list of rooms your house is definitely bigger than ours (for drying inside in bad weather), yet I have never had a problem hanging my clothes to dry. Using energy to dry clothes just doesn’t make any sense for me. From following your blog, I have a feeling you’re on the extreme side of energy conservation (even here in Norway), so I can’t imagine how you must feel in the US. How big do you think the differences are as to the mindset about energy conservation between the US and other countries (e.g Europe)? And why? Is it something inherent in the culture, or is it because of legislations?
The gap in mindset depends on what segment of the U.S. population you ask. Folks like Tom and his readers are probably more conservation minded than the average Norwegian (although I must confess I don’t have a concrete picture of the average Norwegian other than they don’t have a dryer, they live in a (small) apartment, and if they own a car it is also small). I would say that most Americans who can afford a dryer have one. Tom explains reasons why in his reply below.
The (average) American mentality is that you should work 40+ hours per week, save nothing, and consume everything and anything. Any talk of saving energy or reducing waste is seen as a restriction on personal “freedom.” So while the average American is a wage slave AND a debt slave they feel free when they drive down the highway at 80 mph (or 5 mph on their way to work) in their SUV (only 30 payments left!!!).
Culturally speaking, words that are offensive to the average American are: socialist, lazy, poor, cheap/frugal. Words that would not be taken as insults are: wasteful, spendthrift, selfish, reckless. Americans define themselves by their net worth (this is a by-product of capitalism), and since most Americans are not worth what they think they are they make their net worth appear larger by buying trinkets (pea-cocking).
That takes care of the “what” to answer the “why” maybe I should start a blog of my own…
Is there a reason why laundry dryers are so popular in the US? It seems they are the standard way of drying clothes there. Is that true? (I’ve never been to the US.)
Here in Europe (or at least in Germany and Austria, the countries where I’ve lived and which I know well) laundry dryers are seen as kind of an optional household item. Even if you have one – and I would guess 20-30% of households do have one, but I haven’t been able to find any numbers – you generally only use it if you need something dried quickly, or if you don’t have time to hang your laundry on the laundry rack. The conventional wisdom is that dryers cause clothes to shrink and wear out quickly. I don’t know if that’s true or if it’s an urban legend as I’ve never owned a dryer and have never used one in my life.
The usual way of drying clothes here is to put them on a laundry rack after they’ve been spinned in the washing machine. In summer, the rack is put outside (balcony or garden); in winter, it’s put inside which has the nice side effect of providing air humidity (low air humidity is a common cause of respiratory diseases in winter). Don’t Americans do this? I’ve heard putting laundry racks outside is even illegal in some cities!
Also addresses Lars’ comment.
This is certainly an interesting phenomenon. Lots of factors are at play, I think:
Convenience: Americans view the process of hanging clothes to dry a waste of time that could be spent consuming something. Also, not having your pants dry when you need them (at short notice) is considered to be an undue burden.
Status: The prevailing attitude is that poor people don’t have full laundry amenities in their homes. Almost universally, if a home or apartment is outfitted with a washing machine it will be provisioned for a dryer as well. Apartment listings will tout washer/dryer spots, if present.
Aesthetic: Clotheslines are deemed to be unsightly in the U.S. Tied up with status. The condo we rented from 2006-2009 had a rule against hanging clothes in the patio areas. We did it anyway. It would not surprise me if some municipalities had ordinances against clotheslines in an effort to improve the “image” of the community. Even clothes hanging inside would violate aesthetic sensibilities. Americans seem to hold a different standard for foreign countries, where, inexplicably, hanging clothes might even contribute to a quaint charm. I suspect this may stem from a feeling of superiority, at some deeper level.
Prudishness: Having our “smallclothes” on display is uncomfortable for most Americans. I don’t know how universal this is, but my sense is that Europeans care less about who sees their underpants.
In our condo, we stored cat food and cat litter in the dryer, hanging clothes outside—against the rules. We went without a dryer for years at our current place, and even now plan to use it only under mitigating circumstances—part of which relates to health matters/limitations.
Interesting, thanks for the reply. Always good to learn about other cultures. 🙂
Wow that’s fascinating (and sad). Energy conservation sure feels like an uphill battle if using a dryer is considered high-status and convenient!
Thanks for the replies! Andrew C as well.
I will reply for us filthy american pigs. I live in 700 sqft in Chicago. I have a real washer and dryer stacked so that they fit. However, the washer is Energy Star and the dryer has 7 settings. I can go down to a light setting that can dry the most delicate clothing without shrinkage. Before this dryer, I had to lay out most of my “good” shirts since they were to delicate for the Low/Med/High dryer that I had.
Whirlpool, Gas, Duet Steam 9550 – Washer and Dryer. Matching set.
Tom, Why do you take your appliances with you? That is so European. I always leave mine at the place I leave and expect them where I am going.
Gee–it’s never been up to me because the people vacating the place I move into have already decided they are taking their appliances. In this latest move, even the dishwasher, stove/oven and microwave/hood were taken, leaving vacant holes. Craigslist to the rescue!
But … but … consuming things provides jobs for other people in the economy in order to produce those things! That’s how you keep unemployment low! That’s how you grow the economy! You produce things by consuming things! Economic activity doesn’t come from natural resources, it comes from labour working in factories, using their magic fingers and magic technology to turn stuff into goods. [/sarc off]
I never dry my clothes. I use a washing machine but in winter I just hang them up inside overnight and they are good to go by morning, and in summer I can hang them in the sun for an hour and they are dry. This is not only to save energy, but to keep the clothes lasting longer. Look at all the lint you see in the screen in your dryer, well all that stuff came from your clothes!
But then again, people think I’m a bit of a wierdo. I keep my savings in gold and silver, not worthless debt, I must be a kook.
In MY part of the country…
(1) outdoor hanging is impossible, summer or winter, due to precipitation levels
(2) indoor hanging, even after mangling, requires suitable drainage (often not built into laundry rooms), and takes a really long time. Humidity again.
Did nobody at all mention Heat Recovery Ventilators?
Once you have one of those installed — and they run off only a few amps — then you can seal your house up as tight as you like.
People don’t seem to know about them in the US, though. They’re well-understood in Canada, Germany, and Scandanavia.
The passive houses pretty much require heat recovery ventilation – I believe Nicolas’ house has one. The norm for leakiness at 50 Pa is 0.6 1/h for passive houses while the ventilation rate must be at least 0.5 exchanges per hour (Finnish norm, I have no idea what French norm would be), somehow this does not compute without very active ventilation.
I’m also bit curious. If you have such norms for tight houses in US, how the ventilation is supposed to work? If the building is tight, you have to install forced exhaust ventilation, right? So how this saves energy (without heat recovery ventilation)?
i live in finland. during the summer i think almost everybody hangs
their clothes to dry outside. to me it’s really nonsense that someone
in california uses drying machine.
anyway we use energy to keep warm and you use energy to dry and keep cool.
and continue to do so as long as there is energy available.
Tom, you mention that you suspect that the air flow you measured does not fully explain the heating requirements of the house you have also measured. It would be interesting if you did a blog entry on windows. I recently retrofitted all the windows on my house and did a fair bit of research before proceeding. Most people don’t realize how large the heat loss is through glass. It is really a very poor insulator. The window industry has adopted something called U-Factor to rate window performance. It is my understanding that it is just the inverse of R value, probably to obfuscate how bad it is. Accordingly, a typical single pane window has an R value of just 1.5, which barely does more than keep the wind out. I had triple pane windows installed on my house, and even those equate to an R value of just a little more than 5. As a result, I have since made my own Roman style insulated blinds to drop over them at night, and that made more difference than anything else.
I did explore the impact of windows in the thermal house post. Interesting to hear about how much difference your blinds made…
Here in Spain we use “persianas”.This is a roll up exterior up blind made up originally of horizontal wooden slats running in channels, and raised and lowered by a woven nylon strap (mini seatbelt) from inside the window. It rolls up into a compartment above the window within the thicknes of the wall.. The slats are about five centi – sorry, two inches high and about half an inch thick. Each slat has a convex top edge and concave bottom edge. The clever bit is the connectors between the slats.When the blind is partially open ( and hanging from the top) the weight separates the slats by about an eigth of an inch, permitting some light and fresh air to pass through, hence used during hot days to keep out the sunshine. When fully closed (resting on the window cill) the slats are compressed into each other, closing the gaps and forming effective seals. This is your nightime winter position. Modern versions can use plastic coated slats with internal insulation, flexible seals to give really tight closure, insulation in the roller box , and power operation, sometimes remotely controlled by house temperature monitoring equipment. Of course external blinds are far more efficient than internal versions and these things are (at least subjectively) very effective.
In pipe design we use the Darcy-Weisbach method which correlates pressure drop to the square of velocity (or volumetric flow rate).
http://www.engineeringtoolbox.com/darcy-weisbach-equation-d_646.html
That’s a 0.5 exponent which compares to your 0.6 and 0.65. That might be because D-W is for incompressible fluids whereas air is compressible.
The pressure drop comes from both dynamic head (friction) and velocity head (acceleration to get the fluid up to speed). The velocity head difference is v squared over 2g.
glad to see that you haven’t abandoned this blog Tom, I was starting to fear…
Now that you have poured your thoughts out on these pages, can I try to convince you again to also pour out the code used for your figures and calculations? I am particularly interested in the code for the solar power in various regions, since I will need to figure out if it’s worth putting panels on a house that’s built the wrong way around (roof points east and west, no inclined south side).
I’ll add my thanks to the list. Keep ’em coming!
Regarding blower doors, you might find these posts at Green Building Advisors interesting:
http://www.greenbuildingadvisor.com/blogs/dept/building-science/air-leaks-happen-surface-not-volume
and the follow up
http://www.greenbuildingadvisor.com/blogs/dept/building-science/what-s-blower-door-good.
Great post about blower door tests. Here are a couple of hints for likely culprits for your “missing” draft leaks:
In your attic, check not only on the plumbing penetrations and light fixtures (can light and regular – junction boxes generally aren’t well sealed, either), but also plan to seal the tops of your walls where the drywall meets the top plates of the stud walls. Look also for wires that penetrate from wall cavities to the attic – electricians usually use a bigger drill bit than necessary just to make it easier to run wires. Caulk or foam works well, and cuts the chimney draw effect. Plan to air seal your attic thoroughly before blowing in insulation.
Depending on your foundation, that could be a great place to check as well. Crawl spaces are notoriously leaky (often intentionally so due moisture issues or combustion appliances), and sealing between them and the living space can really help. In basements, the plate that bolts into the top of the concrete wall is often not well sealed, and a 1/8″ gap along a whole foundation adds up to major air leakage.
Your comment about baseboard gaps is another good idea. Caulking along trim boards (windows, doors, baseboards, and crown moulding) can usually help find some hidden leaks as well.
from the post:
“it is the interaction of wind with a house that creates pressure differentials inside and outside of the house”
Wouldn’t the indoor-outdoor temperature difference also create pressure differentials? (and yes, I would like you to do the math for me…thanks, love your blog)
Yes, temperature differentials do create pressure gradients. In the Murphy house, however, that effect is probably less significant because they don’t typically use central heating or air conditioning. In houses that do, though, heated air in winter creates a low-pressure zone, drawing outdoor air (and radon gas from the soil if present) into the living space. Chilled air in the summer raises indoor pressure, forcing out all the “bought” air.
The magnitude isn’t large, which is why wind speeds tend to be more important in determining local air infiltration correction factors.